Friday, 14/07/2023, 17:53.
Although I talk a lot how Iron-air batteries are really similar to alkaline hydrogen fuel cells, I didn't look too much on scientific articles building iron-air batteries and neither would ChatGPT, since its data-base is still stuck in 2021 and it constantly says that "metal-air batteries are still in development".
How can it be so sure how these batteries are made if it doesn't even have access to information?
I will try to research a bit on the subject, but I doubt I will bring answers that soon, after all, every paper would make its own iron-air battery on their own way.
Also, sorry for literally just copy pasting a lot of ChatGPT's texts on this Project Log, it is a little lazier than my usual self.
_____________________________________________________________________
Well...
Sh1t, I can't believe I went through all that trouble on researching hydrogen fuel cells and it was all for nothing.
At least, I think, I could use the proton exchange membrane for something else, like a battery.
Not to mention that battery packs are expensive as heck.
The "best" prices I could find on aliexpress was more or less 400 reais (80 dollars) for every kilowatt. I need 18,000 watts per hour, so 180,000 watts in total.
-----------------------------------------------------------------------------------------------------------
I was seriously considering Redux Flow batteries.
Basically, it is more or less just like a fuel cell, just replace the air and hydrogen with two chemical liquids. It is "cheap" and simple to make on a large scale (if you're not using a vanadium-based flow battery).
However, this kind of battery is "only" 10 to 50 watthour per kg, while a lithium ion battery is 100 to 250 watt hour per kg.
That is why it is a great option for grid scale energy storage, unlike lithium ion batteries.
I remember I saw a redux flow battery using lead acid, like car batteries, but as far as I remember, it wasn't that different from conventional car battery.
-----------------------------------------------------------------------------------------------------------
Well, cutting the crap out, one type of battery that is definitely worth making DIY is metal air batteries. These kinds of battery are as energetically dense as gasoline.
For example, Iron air batteries have a *theoretical* energy density of 1200 Wh/kg, so does *theoretically* aluminiun-air batteries with 1000 wh/kg and the highest of all, Lithium air battery with a *theoretical* energy density of 11,000 watthour per kg.
Gasoline has an energy density of 13,000 watthour per kg, however, if you take into consideration the difference between the efficiency of energy conversion of 30% to 40% in combustion engines, then the energy density lowers to 5200 watt hour per kg, while batteries are around 90% to 99% efficient.
So, *theoretically*, I wouldn't need to make a DIY lithium-air battery with 11,000 wh/kg, "just" half of it.
... But, as you could observe, the word "theoretical" was thrown around too much for my taste. These batteries, although have their "theoretical" high number, only achieve around 1000-1500 wh/kg.
One interesting thing is that in the video there is an illustration of an iron-air battery and it is strangely similar to an hydrogen fuel cell, lol
I need to study more about the subject, but I think I can do basically the same thing as I was planning on doing for the hydrogen fuel cell... hmmm.
-----------------------------------------------------------------------------------------------------------
Goddammit, for some reason my brain is not working at all!
I can't focus on anything, my thoughts are a haze of random stuff...
I want to ask on websites like Stack Exchange how to make a Lithium-air battery, but I can't formulate a proper question that doesn't sounds like "hey guys, I don't wanna search/study how it should be done because I'm lazy, so tell me step-by-step how to do it, lol"
-----------------------------------------------------------------------------------------------------------
I asked to ChatGPT about the Lithium-air, Sodium-air and iron-air batteries:
ChatGPT
Lithium-air batteries, also known as lithium-oxygen batteries, are a type of rechargeable battery that utilize oxygen from the air as the cathode reactant. They are still under active research and development, and their commercialization is currently limited. However, I can provide you with information about the electrolytes commonly used in lithium-air battery research.
In lithium-air batteries, the electrolyte typically consists of a lithium salt dissolved in an organic solvent. The choice of electrolyte is crucial for achieving high energy density and overall battery performance. Commonly used lithium salts include lithium bis(trifluoromethanesulfonyl)imide (LiTFSI), lithium perchlorate (LiClO₄), and lithium hexafluorophosphate (LiPF₆). These salts are chosen for their high solubility in organic solvents and compatibility with the other battery components.
As for the organic solvents, researchers often use aprotic solvents such as dimethyl sulfoxide (DMSO), tetraethylene glycol dimethyl ether (TEGDME), or mixtures of these solvents. Aprotic solvents are preferred because they have a wide electrochemical stability window and can provide good lithium-ion transport.
It's important to note that lithium-air battery technology is still in the experimental stage, and there is ongoing research to address various challenges, including the development of more efficient and stable electrolytes. Therefore, the specific choice of electrolyte may vary depending on the research group and the specific objectives of the study.
Iron-air and sodium-air batteries are also types of metal-air batteries that utilize iron and sodium, respectively, as the anode material and oxygen from the air as the cathode reactant. Let's take a look at each of these batteries and the electrolytes used in them:
Iron-Air Batteries:
Iron-air batteries are electrochemical cells that use iron as the anode and oxygen from the air as the cathode to generate electrical energy. Iron reacts with oxygen to form iron oxide (rust) during discharge and is regenerated during the charging process.
In traditional iron-air batteries, the electrolyte used is typically an alkaline solution, commonly potassium hydroxide (KOH) or sodium hydroxide (NaOH). These hydroxide electrolytes facilitate the electrochemical reactions and provide ionic conduction between the anode and cathode. The alkaline electrolyte also helps in controlling the pH and preventing the formation of passivating layers on the anode.
Sodium-Air Batteries:
Sodium-air batteries are similar to lithium-air batteries but use sodium as the anode material instead of lithium. These batteries employ oxygen from the air as the cathode reactant, similar to other metal-air batteries.
Regarding the electrolytes used in sodium-air batteries, the research and development in this area are still ongoing, and various types of electrolytes have been investigated. One approach involves using aprotic organic electrolytes similar to lithium-air batteries, where sodium salts are dissolved in organic solvents. Commonly used sodium salts include sodium bis(trifluoromethanesulfonyl)imide (NaTFSI) and sodium hexafluorophosphate (NaPF₆). Aprotic solvents like DMSO or ethers are often employed.
However, it's worth noting that the development of efficient electrolytes for sodium-air batteries is still an active area of research, and there is no universally established electrolyte system for these batteries yet.
As with lithium-air batteries, it's essential to consult the latest scientific literature and research advancements to stay updated on the specific electrolyte choices and advancements in iron-air and sodium-air batteries.
-----------------------------------------------------------------------------------------------------------
Well, wanting it or not, I think I will be forced to work with Iron-air batteries, since these are the cheapest and the simplest.
I would still literally be using the same materials as an alkaline hydrogen fuel cell.
-----------------------------------------------------------------------------------------------------------
ChatGPT:
"Iron-air batteries consist of several key components, each utilizing specific materials. Here's a breakdown of the materials used in each part of an iron-air battery:
Anode:
The anode in an iron-air battery typically employs pure iron (Fe) or iron powder. The choice between pure iron and iron powder depends on the specific design and requirements of the battery. Pure iron provides a solid metal surface for the electrochemical reactions, while iron powder offers a larger surface area, enhancing the battery's performance.
Cathode:
The cathode in an iron-air battery consists of a porous material that acts as an air electrode. This material allows the necessary oxygen from the air to react with iron during discharge. Commonly used materials for the cathode include carbon-based materials, such as activated carbon, graphite, or carbon black, which provide a conductive matrix for the electrochemical reactions.
Electrolyte:
The electrolyte in an iron-air battery is crucial for facilitating the movement of ions between the anode and cathode during the charging and discharging processes. It enables the flow of current by carrying charged species. Iron-air batteries typically employ an alkaline electrolyte solution, which is a combination of water and potassium hydroxide (KOH) or another alkali metal hydroxide.
Separator:
A separator is used to physically separate the anode and cathode, preventing direct contact while allowing the movement of ions. Commonly, a microporous material such as a polymer membrane is used as a separator in iron-air batteries. The separator needs to be chemically stable and have good ionic conductivity.
Current Collectors:
Current collectors are used to collect the electrical current generated during the battery's operation. These collectors are typically made of conductive materials such as copper or aluminum. They provide a path for the flow of electrons between the battery electrodes and the external circuit.
It's important to note that the specific materials and designs of iron-air batteries may vary based on the intended application and the particular advancements made in the field. Ongoing research and development may introduce new materials or modifications to improve the performance and efficiency of these batteries."
"There are several ways to enhance each part of an iron-air battery by utilizing specific materials. Here are some examples:
Anode Enhancement:
Surface Modification: Coating the pure iron anode with a protective layer, such as iron oxide or iron carbide, can enhance its stability and prevent corrosion during operation.
Alloying: Adding small amounts of other metals to the iron anode, such as nickel or cobalt, can improve its electrochemical performance and durability.
Nanomaterials: Incorporating iron nanoparticles or nanowires can increase the surface area and improve the kinetics of the iron oxidation reactions.
Cathode Enhancement:
Catalysts: Introducing catalysts, such as transition metal oxides (e.g., manganese oxide) or carbon-based materials (e.g., carbon nanotubes), can enhance the oxygen reduction reaction kinetics at the cathode, improving the battery's overall performance.
Porosity Control: Optimizing the pore structure of the cathode material can improve the access and diffusion of oxygen, leading to enhanced discharge rates and capacity.
Electrolyte Enhancement:
Additives: Incorporating additives to the electrolyte, such as conductive polymers or organic compounds, can enhance the ionic conductivity and stability of the electrolyte solution.
pH Control: Adjusting the pH of the electrolyte can impact the battery's performance. Buffering agents or additives can help maintain the desired pH level.
Separator Enhancement:
Ion-Selective Membranes: Implementing ion-selective membranes within the separator can enhance the battery's selectivity, improving its efficiency and preventing unwanted side reactions.
Porous Materials: Using separators with improved porosity and permeability can enhance ion transport and minimize internal resistance.
It's important to note that the selection of specific materials and enhancement techniques may vary depending on the desired performance metrics, cost considerations, and the current state of research and development in the field of iron-air batteries."
"Certainly! Here's a list of enhancing materials for each part of an iron-air battery:
Anode Enhancement:
Iron oxide coating
Iron carbide coating
Nickel alloying
Cobalt alloying
Iron nanoparticles
Iron nanowires
Cathode Enhancement:
Manganese oxide catalyst
Carbon nanotube catalyst
Silver catalyst
Palladium catalyst
Platinum catalyst
Porous carbon material
Electrolyte Enhancement:
Conductive polymers
Organic additives
Lithium hydroxide (LiOH)
Sodium hydroxide (NaOH)
Potassium hydroxide (KOH)
Ionic liquid electrolytes
Separator Enhancement:
Ion-selective membranes (e.g., Nafion)
Microporous polyethylene separator
Polypropylene separator
Ceramic separators (e.g., alumina or zirconia)
Glass fiber separators
Composite separators (e.g., polymer-ceramic hybrid)
It's important to note that the suitability and effectiveness of these materials may vary depending on the specific design and requirements of the iron-air battery. Additionally, ongoing research and development in the field may introduce new materials or modifications that further enhance the performance of iron-air batteries."
"Yes, graphene can be used to enhance the anode of an iron-air battery. Graphene, with its high electrical conductivity and large surface area, can improve the electrode kinetics and stability of the anode.
For cathode enhancement, "porous carbon material" can refer to various carbon-based materials with a porous structure, such as activated carbon, carbon black, or carbon nanotubes. These materials provide a high surface area and facilitate efficient oxygen diffusion and reaction.
Regarding electrolyte enhancement, some examples of conductive polymers that can be used include polypyrrole (PPy), polyaniline (PANI), or poly(3,4-ethylenedioxythiophene) (PEDOT). These polymers can enhance the ionic conductivity of the electrolyte.
The choice of organic additives for electrolyte enhancement depends on the specific requirements of the battery. Examples of organic additives that can be used include organic salts, such as tetraethylammonium tetrafluoroborate (TEABF4) or lithium bis(trifluoromethanesulfonyl)imide (LiTFSI). These additives can improve the stability and conductivity of the electrolyte.
Ionic liquid electrolytes are a type of electrolyte composed entirely of ions in a liquid state at room temperature. Examples of ionic liquids that can be used include 1-ethyl-3-methylimidazolium bis(trifluoromethanesulfonyl)imide (EMIM TFSI) or N-butyl-N-methylpyrrolidinium bis(trifluoromethanesulfonyl)imide (BMP TFSI). Ionic liquid electrolytes offer high conductivity and thermal stability.
Yes, Teflon (polytetrafluoroethylene, PTFE) can be used as a separator material in iron-air batteries. PTFE is chemically inert, electrically insulating, and has good thermal stability, making it suitable for use as a separator material in various battery systems, including iron-air batteries."
"esides lithium hydroxide (LiOH), you can consider using other hydroxides for enhancement in certain battery systems. Some examples include:
Sodium Hydroxide (NaOH): Sodium hydroxide is commonly used in alkaline battery systems as an electrolyte additive or in the construction of alkaline battery electrodes. It can enhance the conductivity and performance of certain battery chemistries.
Potassium Hydroxide (KOH): Potassium hydroxide is another commonly used hydroxide in alkaline battery systems, similar to sodium hydroxide. It can be employed as an electrolyte additive or in electrode materials to improve battery performance.
Barium Hydroxide (Ba(OH)2): Barium hydroxide is utilized in certain types of batteries, such as nickel-iron (Ni-Fe) batteries. It can enhance the electrolyte conductivity and contribute to the overall performance of the battery.
Zinc Hydroxide (Zn(OH)2): Zinc hydroxide is often employed in zinc-based battery systems, such as zinc-air batteries. It plays a crucial role in the zinc electrode reactions and helps maintain the battery's performance.
It's important to note that the choice of hydroxide depends on the specific battery chemistry and design requirements. The selection of the appropriate hydroxide should consider factors such as compatibility, desired performance characteristics, and safety considerations for the particular battery system."
"In the context of an iron-air battery, iron powder can be suspended on a carbon matrix through a process called electrode fabrication. This involves mixing the iron powder with a carbon-based material to create a composite electrode.
Here's a general overview of how iron powder is typically suspended on carbon:
- Selection of Carbon Material: A suitable carbon-based material is chosen, such as carbon black, graphite, or carbon nanotubes. These materials are often chosen for their high conductivity and compatibility with the battery system.
- Mixing: The iron powder and carbon material are mixed together in a proper ratio. This can be done using various techniques, such as ball milling, ultrasonication, or mechanical mixing, to ensure a uniform distribution of iron powder within the carbon matrix.
- Binder Addition: A binder material is often added to the mixture to improve its adhesion and structural integrity. Common binder materials include polymeric substances like polyvinylidene fluoride (PVDF) or carboxymethyl cellulose (CMC).
- Solvent Addition: A solvent, such as N-methyl-2-pyrrolidone (NMP) or water, is added to the mixture to form a slurry. The solvent helps in achieving a suitable consistency for electrode coating or shaping processes.
- Coating or Shaping: The slurry is applied onto a current collector, typically made of conductive material like copper or aluminum foil. Various methods can be used for electrode coating, such as doctor blade coating, tape casting, screen printing, or vacuum filtration. Alternatively, the slurry can be molded into specific shapes using techniques like pressing or extrusion.
- Drying and Compression: The coated or shaped electrode is then dried to remove the solvent, leaving behind the solid electrode material. Compression techniques may be employed to ensure good contact between the iron powder and carbon matrix, enhancing the overall performance.
By suspending iron powder on a carbon-based matrix, the resulting composite electrode benefits from the high electrical conductivity of carbon while utilizing the reactivity of iron during the battery's electrochemical reactions."
-----------------------------------------------------------------------------------------------------------
It is literally the same crap as the alkaline hydrogen fuel cell, lol.
It even needs a Co2 scrubber. Bruh.
Also, assuming that this iron-air battery has 1000 wh/kg, this means that I would need around 180kg of iron powder in order to achieve the 180,000 watts.
Also, I forgot to talk about this, but the way you "charge" the battery is by using a pure hydrogen generator and making that same reduction reaction with iron oxide and hydrogen, were the hydrogen reacts with the oxide, leaving pure iron behind.
The problem is: as you could see in the video, you need the iron oxide and hydrogen to be at high temperatures.
How you would do that in the battery without damaging the other components?
Another thing that kinda bugs me is the maintenence of the potassium hydroxide ion exchange membarne, like shown in the alkaline hydrogen project logs, it uses a membrane soaked with the liquid and it needs to be continiously be in that state.
I'm having some difficulty thinking on how to make the channels to pump the electrolyte without dissolving all the other parts.
Maybe I would need a peristaltic pump (that doesn't need to enter in contact with the liquid), on top of that I think I would add metal or plastic wires through in the alkaline membrane in order to make microchannels that would passively allow for the flow of electrolyte.
-----------------------------------------------------------------------------------------------------------
"To protect the materials in an iron-air battery and mitigate unwanted reactions during charging and discharging, various materials and techniques can be employed for each part of the battery. Here are some examples:
- Anode Protection:
- Coatings: Applying protective coatings, such as iron oxide or iron carbide, on the iron anode can help prevent direct contact with the electrolyte, reducing undesired reactions.
- Passivation Layers: Forming passivation layers on the anode surface can provide a protective barrier, limiting the exposure of the iron to the electrolyte. Passivation can be achieved through controlled oxidation of the anode surface.
- Cathode Protection:
- Protective Layers: Applying protective layers or coatings on the cathode surface can help prevent side reactions or undesired interactions with the electrolyte or ambient air.
- Gas Diffusion Layers: Utilizing gas diffusion layers in the cathode design can promote efficient oxygen diffusion while minimizing undesired reactions.
- Electrolyte Protection:
- Additives: Incorporating additives or inhibitors in the electrolyte solution can help mitigate unwanted reactions or side reactions.
- pH Control: Maintaining the appropriate pH range of the electrolyte can help minimize undesired reactions and optimize battery performance.
- Separator Protection:
- Coatings or Surface Modification: Applying coatings or modifying the surface of the separator material can enhance its stability, chemical resistance, and prevent unwanted interactions with other battery components.
- Functionally Graded Materials: Using functionally graded materials as separators can provide enhanced protection by having varying properties across the thickness of the separator.
It's worth noting that the specific protective materials and techniques may vary depending on the battery design, operating conditions, and the specific challenges faced by the iron-air battery system. Ongoing research and development in the field aim to address these challenges and improve the performance, stability, and safety of iron-air batteries."
Passivation layers are thin protective films that form on the surface of a material to prevent further reactions with the surrounding environment. In the context of an iron-air battery, passivation layers can be formed on the anode surface to protect it from unwanted reactions with the electrolyte.
For passivation layers on the anode in an iron-air battery, materials commonly used include:
- Iron oxides (such as Fe3O4 or Fe2O3)
- Iron carbides (such as Fe3C)
These materials can form stable and protective layers on the anode, preventing direct contact with the electrolyte and minimizing undesired reactions.
For protective coatings on the cathode, various materials can be used, including:
- Polymer coatings (such as polyvinylidene fluoride, PVDF)
- Conductive polymer coatings (such as polyaniline)
- Inorganic coatings (such as thin layers of oxides or nitrides)
These coatings can provide a protective barrier for the cathode, reducing side reactions and enhancing stability.
Gas diffusion layers (GDLs) are typically made of porous and conductive materials that facilitate the movement of gases, particularly oxygen, to the cathode. Common materials used for GDLs include:
- Carbon-based materials (such as carbon paper, carbon cloth, or carbon felt)
- Graphite materials
- Porous metal foams
These materials have high porosity and good electrical conductivity, allowing efficient gas diffusion while providing structural support.
For electrolyte additives, various options can be considered depending on the specific requirements of the battery. Some examples include:
- Conductive salts (such as LiCl or LiTFSI) to enhance the ionic conductivity of the electrolyte.
- Stabilizers or inhibitors (such as additives that reduce side reactions or improve the stability of the electrolyte components) to mitigate undesired reactions.
- Redox mediators (such as ferrocene derivatives) to enhance the electrochemical performance or extend the battery's cycle life.
For pH control in the electrolyte, acid or base substances can be used to adjust the pH level to a desired range. Common materials for pH control in the electrolyte include:
- Acids (such as sulfuric acid or phosphoric acid) to lower the pH.
- Bases (such as potassium hydroxide or sodium hydroxide) to increase the pH.
The specific material and method of using these materials for pH control would depend on the battery design and requirements. It's important to carefully consider the compatibility and concentration of these materials to avoid adverse effects on the battery's performance and stability.
"The ideal pH of an iron-air battery's electrolyte can vary depending on the specific design and requirements of the battery. However, in general, iron-air batteries typically operate in alkaline conditions.
A commonly used pH range for the electrolyte in iron-air batteries is around 13 to 14, which corresponds to highly alkaline conditions. This high pH helps facilitate the desired electrochemical reactions and maintains the stability of the components involved.
The alkaline environment in the electrolyte promotes the reduction of oxygen at the cathode and the oxidation of iron at the anode, allowing for efficient charge and discharge processes. Additionally, the high pH helps mitigate undesired side reactions and provides good conductivity for the ions involved in the battery's operation.
It's important to note that the specific pH range within alkaline conditions may vary depending on the battery design, electrolyte composition, and desired performance metrics. Optimal pH levels can be determined through experimentation and optimization for a particular iron-air battery system."
"If the charging process of the iron-air battery involves a high-temperature reaction between iron oxide and hydrogen, it would indeed require careful consideration to avoid damaging the other components of the battery. Here are a few approaches to address this challenge:
- Segregation of Components: Isolate the high-temperature reaction from the other battery components by physically separating them. This can be achieved by incorporating separate compartments or chambers within the battery design, ensuring that the high-temperature reaction occurs in a dedicated space away from sensitive components.
- Thermal Management: Implement effective thermal management strategies to control and dissipate the heat generated during the high-temperature reaction. This can involve the use of cooling systems, such as heat sinks, fans, or liquid cooling, to maintain the temperature within acceptable limits for the other battery components.
- Heat Shielding: Utilize appropriate insulation or heat shielding materials around the high-temperature reaction zone to minimize heat transfer to the surrounding battery components. These materials should have good thermal resistance and low heat conductivity to protect the adjacent parts.
- Material Selection: Choose materials for the battery components that can withstand the high temperatures involved in the charging process. For example, using heat-resistant materials for separators, current collectors, and other critical components can help prevent damage or degradation.
- Optimal Operating Conditions: Fine-tune the operating conditions of the high-temperature reaction to minimize any adverse impact on the other components. This can involve optimizing parameters such as temperature, pressure, and reaction kinetics to achieve the desired charging process while maintaining the integrity of the battery.
It is important to note that the specific design and engineering considerations would depend on the detailed requirements and constraints of the iron-air battery system. Extensive testing, modeling, and optimization may be required to develop a robust and efficient charging process while ensuring the overall stability and longevity of the battery."
Me:
How can I lower the temperature for the reaction between iron oxide and hydrogen? A catalyst? What kind of catalyst?
-
ChatGPT:
Lowering the temperature for the reaction between iron oxide and hydrogen can be achieved by utilizing catalysts. Catalysts can facilitate the reaction by lowering the activation energy required for the reaction to occur. In the context of the iron oxide and hydrogen reaction, a suitable catalyst can help reduce the necessary reaction temperature.
One example of a catalyst commonly used for this reaction is iron-based catalysts, such as iron nanoparticles or iron-based alloys. These catalysts can enhance the reactivity of iron oxide and hydrogen, promoting the reaction at lower temperatures. Iron-based catalysts have shown promising results in facilitating the reduction of iron oxide by hydrogen.
Several iron-based catalyst alloys can be utilized for the reduction of iron oxide with hydrogen. Some common examples include:
- Iron-Nickel (Fe-Ni) Alloy: Iron-nickel alloys, such as various compositions of Fe-Ni, can serve as effective catalysts for the reduction of iron oxide. These alloys can enhance the reaction kinetics and lower the required reaction temperature.
- Iron-Cobalt (Fe-Co) Alloy: Iron-cobalt alloys, like different compositions of Fe-Co, can also exhibit catalytic activity for the reduction of iron oxide. These alloys offer favorable catalytic properties and can promote the reaction at lower temperatures.
- Iron-Copper (Fe-Cu) Alloy: Iron-copper alloys, such as Fe-Cu alloys, have been explored as catalysts for the reduction of iron oxide. These alloys can enhance the reaction efficiency and provide catalytic activity for the desired hydrogenation process.
- Iron-Palladium (Fe-Pd) Alloy: Iron-palladium alloys, like Fe-Pd alloys, can be utilized as catalysts for the reduction reaction. The presence of palladium in the alloy composition enhances the catalytic activity, enabling efficient reduction of iron oxide with hydrogen.
Other potential catalysts for this reaction include transition metal catalysts, such as nickel (Ni), cobalt (Co), or copper (Cu), or even catalysts based on noble metals like platinum (Pt) or palladium (Pd). These catalysts can improve the reaction kinetics and reduce the required temperature.
The choice of catalyst depends on factors such as the specific iron oxide used, the desired reaction conditions, and the compatibility with the overall battery system. Extensive research and experimentation are typically conducted to identify the most effective catalyst and optimize the reaction conditions for efficient and controlled charging of the iron-air battery.
Creating a carbon matrix at a DIY setup can be challenging and may require specialized equipment and safety precautions. However, I can provide you with a general outline of the process. Keep in mind that producing high-quality carbon matrix materials often involves precise control of temperature, atmosphere, and other parameters, which may be difficult to achieve in a DIY setting.
Materials you will need:
- Carbonaceous material: You can start with a carbon source like activated carbon, charcoal, carbon black, or graphite.
- Binder: A binder material is necessary to hold the carbon particles together. A common choice is polyvinyl alcohol (PVA).
- Solvent: You'll need a solvent to create a slurry with the carbon and binder. Water is a typical choice if you use PVA as the binder.
- Substrate: A suitable substrate, such as filter paper or cloth, where you'll deposit the carbon slurry.
Steps to make the carbon matrix:
- Prepare the slurry: Mix the carbonaceous material (activated carbon, charcoal, etc.) with the binder (PVA) in a suitable ratio. Add the solvent (water) gradually while stirring to achieve a homogenous mixture.
- Apply the slurry to the substrate: Dip or spread the substrate (e.g., filter paper or cloth) into the slurry to coat it evenly with the carbon-binder mixture. You can use a brush or a doctor blade to ensure uniformity.
- Drying: Allow the coated substrate to dry at room temperature or use gentle heat. Make sure the drying process is slow and controlled to avoid cracking or damage.
- Carbonization: Once the slurry is dry, you'll need to carbonize the coated substrate to convert the binder and carbonaceous material into a carbon matrix. This step requires very high temperatures (typically 500°C to 1000°C) and is best done in an inert atmosphere or in a controlled environment to prevent oxidation.
Please note that the carbon matrix produced through this DIY method may not have the same quality and characteristics as commercial carbon matrices used in advanced applications. Advanced carbon materials often require specialized processing techniques and equipment to achieve the desired properties.
If you're looking to use carbon matrix materials for specific applications, it is recommended to source them from reputable suppliers or research institutions that can provide materials with consistent quality and performance. Working with high temperatures and potentially hazardous materials can be dangerous, so always prioritize safety and consider seeking assistance from experts if needed.
In iron-air batteries, the anode is typically made using iron-based materials, and the specific configuration can vary based on the battery design and application. There are several common approaches to fabricating the anode in iron-air batteries:
- Iron Powder Anode: In some iron-air batteries, iron powder is used as the anode material. The iron powder can be mixed with a binder, such as polyvinylidene fluoride (PVDF), to create a paste-like mixture. This mixture is then coated onto a current collector, such as a conductive metal foil or carbon-coated substrate, to form the anode.
- Iron Mesh or Iron Plate Anode: Another common approach is to use an iron mesh or iron plate directly as the anode. In this case, the iron mesh or plate serves as the current collector, and it doesn't require a separate coating process like the iron powder anode. The mesh or plate is often cleaned and prepared to ensure good electrical contact and stability during battery operation.
- Iron Foam Anode: Iron foam is a porous and three-dimensional structure made of interconnected iron filaments. Iron foam can also be used as the anode material in iron-air batteries, providing a larger surface area for electrochemical reactions and potentially improving the battery's performance.
- Iron-Containing Compounds: Some iron-air batteries may use iron-containing compounds as the anode material, such as iron oxides or iron sulfides. These materials can undergo reversible chemical reactions during battery operation, releasing and absorbing oxygen as part of the discharge and charge processes.
It's important to note that the selection of the anode material and configuration depends on various factors, including the specific iron-air battery chemistry, desired performance characteristics, and the intended application. Different approaches may be taken to optimize energy density, charge-discharge rates, and overall battery efficiency.
Additionally, the choice of the anode material can also influence the battery's overall lifespan and cycling stability. Iron-air batteries, like other rechargeable battery technologies, are subject to performance degradation over time, and research focuses on improving cycling stability and overall lifetime.
As the development of iron-air batteries is an ongoing and evolving field, newer materials and manufacturing methods may have emerged since my last update. For the most up-to-date information, it's best to consult recent research literature and battery manufacturers working in the iron-air battery space.
-----------------------------------------------------------------------------------------------------------
Bruh, not even ChatGPT knows how to make the iron-air battery rechargeable.
I would either need to seriously rethink its entire structure for the recharge process or utilizing the goddang platinum that I was trying so hard to scape from so fricking hard.
If the recharging process is structural, meaning that I would literally build the iron-air battery to open up for the reaction to occur, it would be really fricking hard to plan and to build.
I don't even know where to start.
It would need to be constructed like a goddamn disc jukebox...
Maybe without a disc elevator, but inside a giant box that would need to be flooded with hydrogen gas at high temperatures...
-----------------------------------------------------------------------------------------------------------
"While the thermic reduction of iron oxide using carbon monoxide is one of the most common reactions for converting iron oxide into iron, there are other room-temperature reactions that can achieve this conversion. Here are a few examples:
- Hydrogen Reduction: Iron oxide can be reduced to iron by reacting it with hydrogen gas (H₂) at room temperature. The reaction is as follows:
FeO (s) + H₂ (g) → Fe (s) + H₂O (g)
This reaction is commonly employed in certain industrial processes and can be facilitated by using catalysts.
- Aluminum Reduction: Iron oxide can be reduced to iron by reacting it with aluminum metal (Al) at room temperature. The reaction is highly exothermic and requires careful handling:
Fe₂O₃ (s) + 2Al (s) → 2Fe (s) + Al₂O₃ (s)
This reaction is commonly known as the thermite reaction and is often used for welding and metal casting.
- Biological Reduction: Some microorganisms, such as certain bacteria, are capable of reducing iron oxide to iron under specific conditions. This biological reduction can occur at room temperature and is often associated with processes like microbial corrosion or biomineralization.
It's important to note that while these reactions can, in principle, occur at room temperature, they may require specific conditions, such as the presence of catalysts or microorganisms, to proceed at a reasonable rate. In industrial settings, elevated temperatures are often employed to accelerate the reduction process."
"Biological reduction of iron oxide to iron typically occurs under specific environmental conditions that are favorable for the growth and metabolism of certain microorganisms. These conditions can vary depending on the specific bacteria involved, but some general factors that promote biological reduction include:
- Anaerobic Environment: Biological reduction of iron oxide to iron usually occurs in environments devoid of oxygen or with low oxygen concentrations. Oxygen inhibits the activity of iron-reducing bacteria, so anaerobic conditions are necessary for the reaction to take place. This can be achieved in sediments, groundwater, or other oxygen-limited environments.
- Presence of Electron Donors: Iron-reducing bacteria require a source of electrons to drive the reduction reaction. Common electron donors used by these bacteria include organic compounds such as acetate, lactate, or other short-chain fatty acids. These compounds are metabolized by the bacteria, releasing electrons that are used in the reduction process.
- Availability of Iron Oxide: Iron-reducing bacteria require a source of iron oxide to carry out the reduction reaction. This can be naturally occurring iron oxide minerals in the environment or anthropogenic sources of iron oxide.
- Suitable pH and Temperature: Iron-reducing bacteria have specific pH and temperature ranges within which they can thrive. These ranges can vary depending on the bacterial species involved but are typically in the neutral to slightly acidic pH range (around 6.5 to 7.5) and moderate temperatures.
- Nutrient Availability: Like other microorganisms, iron-reducing bacteria require essential nutrients such as nitrogen, phosphorus, and trace metals for their growth and metabolism. Adequate availability of these nutrients is necessary for the biological reduction process.
It's important to note that the biological reduction of iron oxide by microorganisms is a complex process influenced by various factors, including the specific microbial community, environmental conditions, and the availability of electron donors and acceptors. The efficiency and rates of biological reduction can vary depending on these factors, and the reaction may occur over extended periods of time."
-----------------------------------------------------------------------------------------------------------
Well, it would be nice to have a battery that self recharges using modified microorganisms...
I think I found a good solution for the recharging process using hydrogen gas to convert iron oxide back to pure iron.
The iron powder would need to be suspended in another ion-exchange membrane, like an iron-gel composite, and once the the battery is fully discharged, and thus, the iron powder fully converted in iron oxide powder, you would need to insert hydrogen gas in the air electrode.
The electrolyte gel would act like an ion-exchange membrane, allowing the transportation of the hydrogen to the iron powder, which would react with Iron oxide, making pure iron and water.
Since the entire thing is a gel, it would then form blobs of water that would (probably) dissolve into the fluids of the electrolyte fluid that also pumps around the ion-exchange membrane.
I believe that an iron-air battery exactly like an alkaline hydrogen fuel cell (with cascade slots) would ocuppy too much space, I think you would still need to use the swiss roll battery style for the entire thing. Just like a conventional baterry.
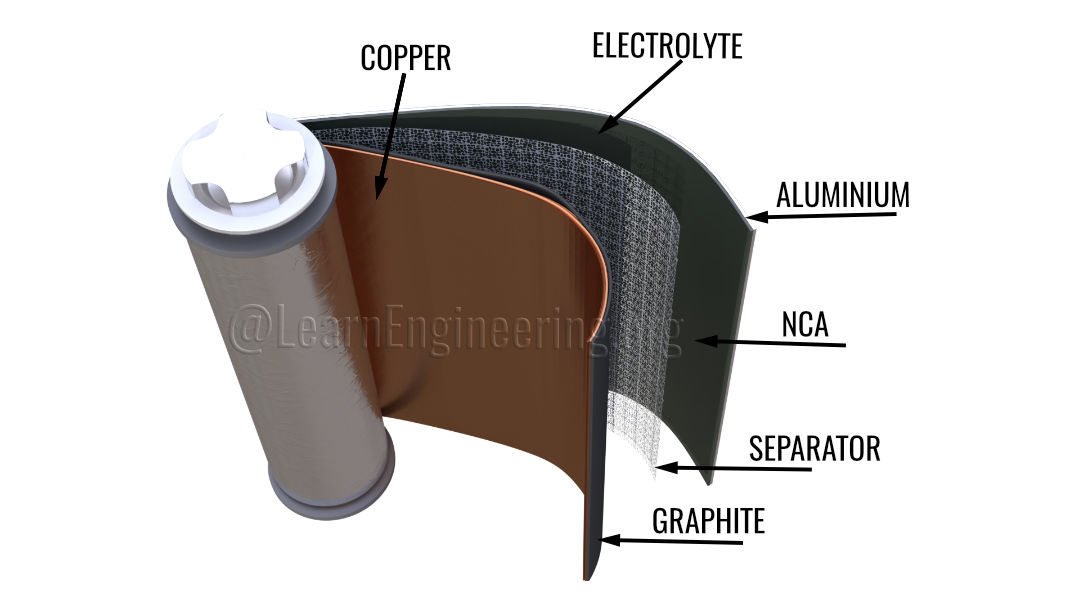

Although apparently simpler, I don't know how to do such thing in a DIY setup...
Again, "probably", I don't yet know if it would work, but at least it is an interesting option to work from, since, you know, I don't have any other options left.
-----------------------------------------------------------------------------------------------------------
"I apologize for the confusion. To further enhance the efficiency and reliability of an iron-air battery, here are additional suggestions for enhancers or protective layers:
- Oxygen Reduction Catalyst: Implement an efficient catalyst at the cathode to enhance the oxygen reduction reaction. Materials such as platinum (Pt), palladium (Pd), or other transition metal-based catalysts can improve the cathode performance and increase overall battery efficiency.
- Oxygen Evolution Catalyst: Utilize a catalyst at the anode to promote the oxygen evolution reaction. Suitable catalysts, such as metal oxides or perovskite materials, can enhance the anode kinetics and reduce overpotential during charging.
- Surface Coatings: Apply protective coatings on the electrode surfaces to prevent undesired reactions or side reactions with the electrolyte. Examples include thin films of polymers, oxides, or protective layers formed through chemical or electrochemical deposition.
- Passivation Layers: Create passivation layers on the anode and cathode surfaces to protect against degradation and unwanted reactions. These layers can be formed through controlled oxidation or through the application of protective films.
- Ion-Selective Membranes: Integrate ion-selective membranes within the separator to enhance ion transport and prevent unwanted species crossover between the anode and cathode. These membranes can improve battery efficiency and reduce the risk of side reactions.
- Composite Electrodes: Develop composite electrodes by combining iron-based materials with other compounds or materials. This approach can improve the performance, stability, and conductivity of the electrodes.
- Advanced Electrolyte Formulations: Explore advanced electrolyte formulations that incorporate additives or functionalized salts to improve ionic conductivity, stability, and suppression of side reactions. These formulations can enhance battery performance and extend cycle life.
- Separator Coatings: Apply coatings or surface modifications to the separator material to enhance its stability, electrochemical performance, and resistance to degradation. These coatings can also prevent unwanted reactions with other battery components.
Implementing these enhancers or protective layers can help improve the efficiency, stability, and overall performance of the iron-air battery, reducing potential failings and enhancing its longevity. It's important to note that the specific choice of materials and methods would depend on the battery design and optimization through experimentation and testing."
"Certainly! Here are a few more suggestions to enhance the efficiency and reliability of an iron-air battery:
- Porous Electrodes: Design the anode and cathode with a porous structure to increase the surface area and promote efficient gas and ion diffusion. This can enhance the battery's performance and overall energy efficiency.
- Advanced Current Collectors: Utilize high-performance current collectors, such as conductive carbon-based materials or metal foams, to improve current collection and reduce resistance within the battery system.
- Redox Mediators: Incorporate redox mediators in the electrolyte to facilitate electron transfer and enhance the battery's electrochemical reactions. Redox mediators can improve the battery's efficiency and cycling stability.
- Active Material Modifications: Explore surface modifications or doping techniques to enhance the properties and performance of the active materials used in the anode and cathode. This can improve their reactivity, stability, and cyclability.
- Gas Diffusion Enhancers: Introduce additives or materials that enhance the diffusion of oxygen or other gases at the cathode, such as mesoporous materials, to improve the battery's overall performance.
- Microfluidic Systems: Implement microfluidic systems within the battery design to improve reactant distribution, promote uniform reactions, and minimize concentration gradients. This can enhance the battery's efficiency and reduce the occurrence of localized cell imbalances.
- Self-Healing Mechanisms: Explore the incorporation of self-healing materials or mechanisms within the battery system to repair any physical or chemical damage, improving the battery's longevity and reliability.
- Electrode Surface Modification: Apply surface coatings or functionalize the electrode surfaces to enhance their stability, reduce degradation, and promote efficient charge and discharge processes.
- System-level Optimization: Optimize the overall battery system through system-level design considerations, such as balancing component sizes, optimizing electrode/electrolyte thickness ratios, and carefully designing the cell geometry to maximize performance and efficiency.
Each of these enhancements requires thorough research, development, and testing to ensure their compatibility and effectiveness in the specific iron-air battery system. Continuous innovation and optimization can lead to further improvements in the efficiency, reliability, and overall performance of iron-air batteries."
"A redox mediator is a compound that facilitates electron transfer between the active materials in a battery. It acts as a shuttle, helping to transport electrons between the anode and cathode, thereby improving the overall efficiency and performance of the battery.
Various substances can be used as redox mediators, depending on the specific battery chemistry and design. Some examples include:
- Quinones: Compounds like anthraquinone, benzoquinone, or hydroquinone can serve as redox mediators in certain battery systems.
- Organic Molecules: Organic compounds, such as viologens (e.g., methyl viologen) or ferrocene derivatives, can act as redox mediators due to their reversible redox behavior.
- Transition Metal Complexes: Complexes of transition metals, like iron, cobalt, or copper, can function as redox mediators by undergoing reversible redox reactions.
Doping a material involves introducing impurities or foreign atoms into the crystal lattice of a host material to modify its properties. To dope a material in a DIY setup, you would typically need to acquire the desired dopant compound and incorporate it into the material during its synthesis or fabrication process. The specific material and dopant will depend on your objectives and the desired properties you aim to achieve.
Creating mesoporous materials in a DIY setup can be challenging as it typically involves specialized techniques and equipment. However, one approach you can consider is using a template-based method. This involves mixing a precursor material with a template, such as micelles or colloidal particles, and subsequently removing the template to leave behind the desired mesoporous structure. Extensive research and experimentation are usually required to optimize the synthesis conditions and obtain well-defined mesoporous materials.
For self-healing mechanisms, one approach is to incorporate microcapsules filled with healing agents into the electrode or separator materials. When damage occurs, the microcapsules rupture, releasing the healing agents that can repair the damage by filling in gaps or restoring the integrity of the material. The selection of suitable healing agents will depend on the material being used and the nature of the damage.
Electrode surface modification can be achieved through various methods, including:
- Chemical Vapor Deposition (CVD): Deposition of a thin layer of desired materials onto the electrode surface using chemical reactions in a controlled environment.
- Physical Vapor Deposition (PVD): Deposition of materials through processes such as sputtering or evaporation in a vacuum environment.
- Electrochemical Methods: Utilizing electrochemical techniques like electrodeposition or electrochemical polymerization to modify the surface of the electrode.
- Wet Chemical Methods: Immersing the electrode in a solution containing suitable materials or precursors to achieve surface modification through chemical reactions.
The specific materials used for electrode surface modification will depend on the desired objectives, such as improving stability, enhancing conductivity, or facilitating specific electrochemical reactions. Examples include conductive polymers, metal oxides, or protective coatings like graphene or carbon nanotubes.
It's important to note that advanced techniques and equipment are often required for successful material doping, mesoporous material synthesis, self-healing mechanisms, and precise electrode surface modification. If you are considering these approaches, it's advisable to consult scientific literature, seek expert guidance, or collaborate with researchers familiar with these techniques to ensure safe and effective implementation."
Also, I think I found a way of making these thousandas of membranes for the iron-air battery.
Basically, the biggest concern I had was with the ion-exchange membrane, I didn't had any idea on how to make it in bigger quantities and then impregnate all of the membranes with KOH.
Basically, the idea is to simply follow that tutorial I posted, but instead of inserting the membrane on the Crosslink fluid and then on the alkaline fluid, you "just" stack the membrane on the battery.
And then, since the battery will supposedly have a liquid circulation system for the electrolyte, you can just pump the Crosslink fluid, extract it and then finally pump the electrolyte in order to impregnate the membranes.
This would be way more practical than wait x amount of hours for every step.But you would still need to find a way of heat it up to 60ºC just like in the tutorial.
(this one, the others are just for anyone who wants a different type)
Also, I think you could instead of inserting pure iron powder on the membrane, insert instead iron oxide and once everything is ready, connect the hydrogen generator to the air port and "recharge" the battery by converting the iron oxide inside the membrane into pure iron, resulting in more water on the membrane.
Since you need sodium hydroxide/potassium hydroxide to make an hydrogen generator, the full battery cycle is selfcontained.
I *think* that, since the oxygen generated by the division of water into pure hydrogen will be used to transform iron oxide into pure iron, the oxygen gas left in the chamber would be used to convert iron into iron oxide. Generating electricity.
But I'm not so sure on how exactly one would avoid the automatic reaction of iron with the oxygen in the chamber/battery.
The whole idea of a self-cointained iron-air battery would be to avoid the use of a Co2 scrubber, but I don't know it would work.
By the way, again, let's remember how to generate pure hydrogen and pure oxygen in different chambers:
The only thing that I don't like is how the hydrogen and oxygen are only separated by gravity/water, any tilting on the equipment and you have yourself in a kaboom situation.
Also, this device takes 25 minutes to make 1 galloon of hydrogen, if you were to react with the 200kg of iron oxide powder in the fuel cells, you would need around 22 gallons, which would take 9 hours to recharge the battery with only one hydrogen generator.
So, you would need around 9 of those to reduce the time for recharging to one hour, I suppose.
This one is in brazilian portuguese, but you can translate the subtitles on youtube, and on this one, the hydrogen and oxygen are separated in different chambers.
The other videos are just other options and/or perspectives on the subject.
----------------------------------------------------------------------------------------------------------
Also, I was trying to think on how the actual frick I will store and/or manipulate the iron powder (or just the metal powder in general) without literally rusting everything.
Even at ambient conditions, the iron oxide powder rapidly reacts with the air.
Of course, I could just ignore it for the iron-air battery, since it can revert the iron oxide back into iron during recharging, but I can't do that for the stator of the electric motors, that need pure iron powder.
I would need to find a way of making a vacuum glove box so I can work with everything without the risk of messing up its elements.
Oh, by the way, I think this is how you make carbon black/carbon vulcan/conductive carbon for Fuel Cells and/or Batteries (the guy made a full series on the subject):
By the way, I was thinking on using glitter sprayer for cakes as the sprayer for both the Iron-Air battery electrodes and the electric motor soft magnetic composite stator.
Discussions
Become a Hackaday.io Member
Create an account to leave a comment. Already have an account? Log In.